Brain Cancer: How Columbia Researchers Are Fighting Back

Last summer, U.S. Sen. John McCain (R-AZ) was diagnosed with glioblastoma, and his ongoing treatment has drawn new attention to brain cancer. Approximately 23,000 Americans are diagnosed with malignant brain cancers annually. Though far from the most common form of cancer—lung cancer afflicts more than 200,000 Americans each year—brain cancers are uniquely difficult to treat.
Glioblastoma, the kind of cancer McCain has, is by far the most common form of malignant brain tumor. The risk of glioblastoma increases with age and is higher in men than women, but researchers have few clues about what causes the disease.
What is clear is that it's an aggressive form of cancer. Less than 10 percent of patients with glioblastoma multiforme survive more than five years after diagnosis. That figure has not changed much in the past 30 years, despite dozens of clinical trials. Researchers are far from giving up, though. At Columbia University Irving Medical Center and Herbert Irving Comprehensive Cancer Center, a multidisciplinary team of basic scientists, translational researchers, and clinicians is working on multiple fronts to advance this field.
How do you look for new treatments?
Traditionally, the search for new brain cancer treatments has been somewhat haphazard, with scientists testing huge numbers of compounds against cultured tumor cells and animal models of the disease and hoping for a few lucky hits. Though that sometimes works, researchers are now building the tools for a more targeted strategy.
For example, Anna Lasorella, MD, deliberately keeps tumors alive—in laboratory dishes. "We isolate cells from tumors that are operated on at Columbia and other institutions and grow the cells in a particular 3-D condition so that they can represent the tumor from which they are derived," says Lasorella. The lab's library of miniature tumors lets researchers probe the biology of brain cancer and also test potential drugs against it.

Columbia researcher Anna Lasorella makes 3D cultures of brain tumors to look for new ways to treat the cancer. Image: Anna Lasorella/CUIMC
In one recent project, Lasorella and her colleagues identified a crucial change inside tumor cells, which helps them proliferate and recruit new blood vessels to feed themselves. The researchers are now building computer algorithms to identify the chemical compounds most likely to disable this mechanism.
After identifying promising compounds, the team can then return to cultured tumors to test the compounds. Lasorella says the 3-D cultures do a much better job than traditional two-dimensional cell cultures when it comes to modeling what a drug will actually encounter in a patient. The drug "has to cross several layers to reach the core of the sphere, which mimics more the diffusion that a drug has to have in the real brain tumor," says Lasorella. The tumor spheres can also be injected into mouse brains, allowing the researchers to study their drug candidates in live animals.
What are the challenges in studying brain cancer?
Everything currently known about brain tumors comes from the material removed at the time of surgery. Many brain cancer labs around the world use these samples to search for molecular changes that could be targeted to slow the tumor’s spread.
"The challenge is that tumors are very heterogeneous,” says pathologist Peter Canoll, MD, PhD.
The core of the tumor is composed of densely packed glioma cells, and surgeons usually try to remove the entire core.
“But the area of brain tissue surrounding the tumor core is diffusely infiltrated with cancer cells,” Canoll says. Surgeons can’t remove the entire area of infiltration, so many cancer cells are left behind in the brain. These residual cancer cells almost always give rise to recurrent tumors months or years after surgery.
“If the cells we’re looking at in the lab don’t resemble the cells left behind in the brain, we may not be identifying the right targets.”
In an effort led by Jeffrey Bruce, MD, surgeons at Columbia are now removing samples from these margins, and Canoll and collaborator Peter Sims, PhD, have been comparing them to cells from the tumor’s core. Using newly developed techniques, they examine each cell individually to get a better idea of the kinds of malignant cells left in the brain after surgery.
One difference is already clear: The cells can crawl.

“The cancer cells in margins intermingle with normal neurons, astrocytes, and other types of brain cells,” Canoll says, “and to spread, they need to squeeze through the tiny spaces in between.”
Canoll and other researchers have learned that the cells use certain molecular motors to wriggle their way through a thicket of brain tissue, so the researchers are using animal models and cell cultures to look for ways to jam the motors.
“With single-cell sequencing, we’re seeing that cells respond differently to this strategy,” he says. “Some cells die and disappear. But others change and become resistant. We will probably need a cocktail of drugs that can overcome that resistance.”
How many types of brain cancer are there, and will there ever be a "silver bullet" to treat them all?
Doctors currently categorize brain cancers based on the tumor's location and the specific types of cells involved. This has produced a taxonomy of about 20 different types of brain cancers. In recent years, though, researchers have discovered subtle but critical differences within these groupings, leading to more sub-types.
In fact, ongoing projects such as the National Institutes of Health's Cancer Genome Atlas suggest that there may be hundreds, if not thousands of distinct forms of the disease. "What we know now is that every single tumor is different from each other," says Antonio Iavarone, MD. That's bad news for anyone hoping for a "silver bullet" that will treat all types of brain cancer, but it points the way to a different sort of treatment.
By sequencing the DNA and RNA of tumors, Iavarone and his colleagues can now reveal the specific defects underlying each patient's cancer. In one recent project, the team discovered an alteration in some tumors that welds two different proteins together. This "gene fusion," present in about 3 percent of human glioblastomas, appears to be a critical driver of tumor growth. Chemicals that target the fusion can effectively treat this sub-type of glioblastomas in a mouse model of the disease.
Already, two clinical trials are underway testing the compounds in cancer patients. Physicians in France are trialing an experimental compound that targets the fusion in brain cancer patients who express it. In the United States, another experimental drug that targets the fusion is being tested in patients with brain, urothelial, and other cancers.
The new work is part of a major trend toward "precision medicine," in which sophisticated gene sequencing and data processing will allow doctors to tailor individualized treatments for each patient. It's a powerful idea, but a hard one to implement. "The therapeutic effects are very strong, however the identification of this fusion is complicated and requires very sophisticated analysis, so this is not necessarily done in the clinic," says Iavarone. He adds that "there is currently a notable gap between what's happening in basic science and what happens in a clinical setting."
Have any new brain cancer treatments made it into the clinic recently?
Despite the hurdles, numerous clinical trials are ongoing at major brain cancer centers. These trials are tackling the biggest challenges associated with treating tumors in the brain. There are "a couple of problems with brain tumors: Not only do they grow very quickly, but all the cells within the tumor are often different from each other, so treatments may be able to kill most of the tumor cells but generally can't kill all of them," says neurosurgeon Jeffrey Bruce, MD.
Tumor cells that survive initial treatment can grow into new tumors that are harder to treat. Even when researchers have found effective drugs that can kill these recurrent growths, the body's natural barrier between the brain and bloodstream makes it hard to deliver them to the tumor.
To address that, Bruce and his colleagues have developed a system that drips chemotherapy drugs directly into the brain.
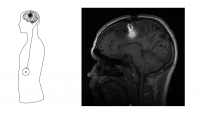
A pump implanted in a patient can deliver chemotherapy directly to the brain. Image: Jeffrey Bruce / CUIMC.
"You take a simple thin catheter and you can surgically implant it right into the center of the tumor," says Bruce. The first version of the system fed drugs into the catheter from an external pump, but now the team can implant the pump into a patient's abdomen, permitting longer treatments while reducing the risk of bacterial infection. The next step is to combine the catheter delivery system with customized targeting of a patient's specific tumor cells.
For patients who have been diagnosed with brain cancer, Bruce has two pieces of advice. "I urge them to be treated at a major medical center with a brain tumor center that's trying new things," says Bruce and "although the prognosis for these tumors is limited, we just encourage people to continue to live their lives as fully as possible."
###
Anna Lasorella, MD, is a professor in the Departments of Pathology & Cell Biology and Pediatrics at Columbia University Vagelos College of Physicians and Surgeons.
Peter Canoll, MD, PhD, is director of neuropathology and professor of pathology & cell biology at the Vagelos College of Physicians and Surgeons.
Peter Sims, MD, is assistant professor of systems biology at the Vagelos College of Physicians and Surgeons and associate director of the JP Sulzberger Columbia Genome Center.
Antonio Iavarone, MD, is a professor in the Departments of Pathology & Cell Biology and Neurology at the Vagelos College of Physicians and Surgeons.
Jeffrey Bruce, MD, is the Edgar M. Housepian Professor of Neurological Surgery at the Vagelos College of Physicians and Surgeons; director of the Bartoli Brain Tumor Research Laboratory; and co-director of the Brain Tumor Center.